Harvesting sunlight to fuel the modern world
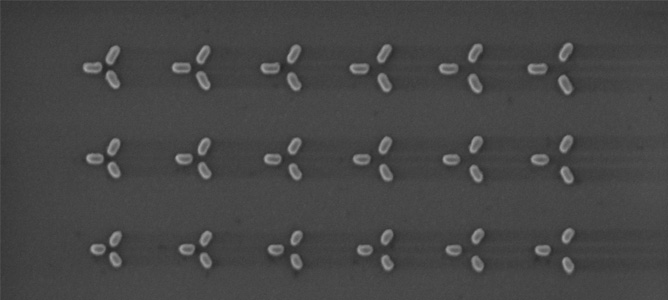
A Scanning Electron Microscope (SEM) image of a set of nanoparticles designed for capturing light. The basic design consists of an isosceles triangle of nanorods. Each nanorod is made of gold. By changing the sizes and separation among the nanorods, we can control which part of the solar spectrum get trapped by the nanoparticle trimer.
March 2013
Harvesting energy from light sources, including the sun, could provide a significant percentage of the world’s energy needs over the next century. In order to meet this critical demand, it is crucial to devise ways to transform light energy into chemical fuels, not just electricity. The aim of this project is to create new nanostructures that directly couple light energy into chemical reactions. In this study, researchers from CSIRO and the University of Melbourne, led by MCN Technolgy Fellow Dr. Daniel Gomez, performed the first step in this process - capturing and storing light energy with a system of nanoparticles.
The team created a system of nanoparticles that captures a significant amount of light which is able to store this energy for longer than most traditional systems. This feat is achieved by suppressing “re-radiation of light” by using metal nanoparticles that do not efficiently radiate light. The basic design consists of three metallic nanorods arranged in the shape of an isosceles triangle.
The metal nanoparticles were firstly designed at the CSIRO and The University of Melbourne using a sophisticated but simple theoretical model. The design was later transformed into real nanoparticles at MCN and was later put into action by testing their optical properties at the Optics laboratories at the University of Melbourne.
The nanoparticles were created using the electron beam lithography tool in the MCN cleanroom in addition to high resolution imaging equipment (scanning electron microscope), optical characterisation equipment (spectroscopic ellipsometer) and advanced metal processing tools (electron beam evaporator).
The most significant aspect of this project is that the team have achieved light trapping at the nanoscale by design. Starting with a highly sophisticated theoretical model of nanoparticles, they were able to create a structure that was able to interact with light (of particular wavelength and polarisation) and trap it more efficiently than most common systems. No single publication to date comprises a set of experimental and theoretical results as consistent and homogenous as the one presented by the group on the subject of ‘dark plasmonic modes.’ Due to the experimental precision, the excellent qualitative agreement between experimental data and the theoretical model and the large parameter space investigated, their study is a strong first step in the greater goal of creating solar fuels.
This study leads the way in a succession required for demonstrating solar-to-chemical energy conversion. While the general public often associates solar energy to the generation of electricity, sunlight can also be used for driving important chemical reactions such as the production of solar fuels. Some of these fuels include hydrogen gas, whose only product after combustion is water (not even a small trace of CO2, thus a fully carbon emission free technology). The outcomes of this grand challenge, namely using nanotechnology for solar-to-chemical energy conversion, will lead to low emissions, renewable energy sources and to a future Australian nanomaterial manufacturing industry.
After capturing light, the next step is to devise ways to do useful work with the energy trapped from it. The team at MCN will incorporate the nanoparticle designs into advanced architectures to demonstrate key chemical reactions driven entirely by sunlight.