Electronic skins – the future for medical devices
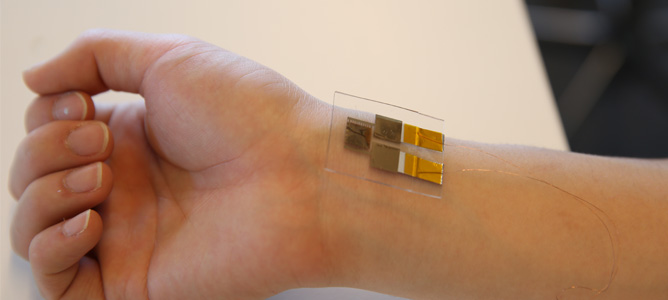
A sample of the wearable sensor.
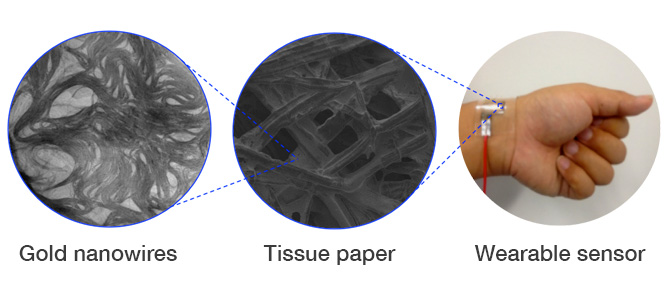
The impregnation of tissue papers by ultrathin gold nanowire inks lead to highly-sensitive and wearable pressure sensors.
July 2014
Technologies are increasingly becoming smaller, lighter and smarter, and now they are becoming flexible and wearable too. Current rigid circuit board technologies are limited in their applications, particularly with regards to medical devices and bionics. MCN Technology Fellow, Professor Wenlong Cheng from Monash University, has been leading a team in the development of stretchable, elastomeric conductors for use in pressure sensing.
Electronics such as these which are soft, flexible, stretchable and wearable are enabling applications that are impossible to achieve with circuit board technologies. The focus of this project is the creation of pressure sensors which enable portable pulse and heart rate monitoring, as well as body movement sensing.
These sensors are not achievable with traditional organic or inorganic materials or conventional manufacturing methods. Utilising the facilities at MCN, Professor Cheng’s team have developed a simple yet efficient and low-cost nanotechnological approach to integrating ultrathin gold nanowires into tissue paper to create an ultra-flexible sensor. This can then be sandwiched between thin layers of PDMS and attached to wiring to create a wearable sensor that can provide readings of blood pressure, heart rate and movement. Such sensors are so sensitive that tiny forces from blood pulses and acoustic vibrational forces can be accurately detected.
This development of soft electronics holds huge potential for portable health monitoring devices. Professor Cheng will be looking at the commercial development of this project next.
Professor Cheng can be heard discussing these sensors on the BBC radio program, Click. The interview starts approximately 6 minutes into the show.
You can read more about this project in the Nature Communications Paper, A wearable and highly sensitive pressure sensor with ultrathin gold nano wires, published in February 2014.
Nanoscale optical circuits for light-speed information processing

An example of a nanoscale optical circuit that measures optical phase difference. a) A graphic of the circuit which consists of three gold nanorods supporting localised surface plasmon resonances. One of the nanorods outputs an optical signal proportional to the difference between the two inputs. b) An SEM image of the resist pattern on a gold film prior to etching.

c) A microscope image of a circuit when the optical phase difference across the inputs is zero. The circuit cannot be seen because there is no output for zero phase difference. d) A microscope image of a single circuit when the phase difference is 40o. The circuit is too small to resolve but the light output from it is easily observed.

Two examples of nano-plasmonic circuits based on evanescent coupling between localised surface plasmons that show the circuit diagrams and their representations as metal nanorods.
April 2014
Modern communications networks that support the internet rely on light waves to carry information. In these systems, digital signals are imposed on the light beams, which travel long distances on optical fibres.
Currently these systems rely on electronic devices to encode and decode the information. While electronics can be integrated into high-density circuits, the limited speed at which these circuits function limits the amount of data that can be encoded on the light beam. Conversely, optical circuits with much faster speeds can be created using optical waveguides integrated into silicon chips, however they cannot achieve the same density as electrical circuits.
Led by MCN Technology Fellow Dr. Tim Davis, researchers from CSIRO have been utilising the MCN facilities to develop nano-scale optical circuits which operate at exceptionally high speeds and which can be integrated into high-density circuits. These ultimately demonstrate optical information processing.
The circuits are based on configurations of gold nanorods that support localised surface plasmon resonances – electric charge oscillations that are excited by light on metal surfaces. The team fabricated an optical circuit that was barely 200nm wide which measured the phase difference of an incident light wave across its inputs.
The circuit has many applications including the demodulation of optical signals with information encoded by phase shift keying. These nanoscale circuits operate extremely quickly and are able to respond to signals thousands of times faster than conventional electronic circuits, at a rate of up to 50,000 GHz.
To achieve these extremely fast nanoscale circuits, the team utilised the Electron Beam Lithography (EBL) tool at MCN. A glass wafer was first covered in a thin layer of gold about 30nm thick, after which an electron beam sensitive resist was coated over the gold. This was then exposed by the EBL. After the resist was developed, the circuits were imaged in the scanning electron microscope to check the integrity of the fabrication. The final step was to etch the gold film in the ion-etching instrument, which removes the gold unprotected by the resist. The result is a set of metal nano-rods that form the optical circuit.
While the optical circuits are too small to be imaged in an optical microscope, it is still possible to see the light scattered from them. The team was able to show that a single circuit could output an optical signal related to the phase difference of the light wave incident at its inputs. Incredibly, the entire device is about one quarter of the wavelength of light and probes the optical phase difference within a small fraction of a wavelength. This can be likened to a nano-scale voltmeter probing the electric field of the light wave.
The measurement of optical phase can be viewed as a form of optical signal processing. It is possible to show that optical circuits based on localised surface plasmons can perform a variety of linear mathematical operations between optical signals, all at the nano-scale. This project demonstrates that optical circuits of this type are feasible and there are a large number of possible circuits that can be designed, providing a means for processing information encoded on light beams.
The next steps in the project are to develop a range of other optical circuits and combine them. The team has already shown that arrays of optical circuits can be used to create a metamateria which enables the modulation and switching of one light beam by another. They will also be creating artificial materials based on these circuits that will be used for image processing.
You can read more about this project in Measuring subwavelength phase differences with a plasmonic circuit—an example of nanoscale optical signal processing, published in Optics Info Base.
Metal nanoparticles lead the way towards solar water decontamination
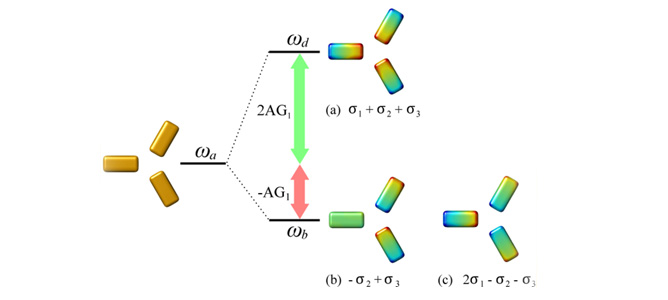
Three lowest-order modes of an interacting triangle of particles. Mode a) is the highest in energy of the three and is a mode with radial symmetry. Modes b) and c) are degenerate modes which are characterised by having a net dipole moment and perpendicular polarisations.
April 2014
It has long been known that solar energy can be used to create electric energy for heating and lighting. It can also be used however, to drive important chemical transformations such as the production of renewable chemical fuels by extracting hydrogen from water and hydrocarbons from carbon dioxide. Other uses include the sequestration of air pollutants such as greenhouse gases, low-cost water purification for developing countries, and the low carbon-footprint production of fine chemicals such as disinfectants and self–cleaning surfaces. There are countless other as yet unforeseen applications of solar to chemical energy transformations.
Existing approaches for harnessing solar energy for these applications are relatively inefficient and therefore not widespread. In order to improve the efficiency of solar-to-chemical energy conversion processes, it is important to maximise the light collection efficiency.
In this project, researchers from CSIRO led by MCN Technology Fellow Dr. Daniel Gomez, are creating novel metal nanoparticles capable of efficient light collection and focusing, in order to enhance the efficiency of light-driven chemical reactions. Utilising the Electron Beam Lithography (EBL) system, thin film deposition and characterisation tools available at MCN, the team have created networks of precisely controlled metal nanoparticles with precision down to 10nm.
Preliminary results indicate that certain nanoparticle arrangements can lead to an enhancement of light-driven decomposition of water pollutants by at least eight times the current rate of decomposition. They have achieved this by creating arrays of nanoparticles using aluminium, an abundant and inexpensive metal, which offers great potential for future deployment in large-scale applications.
The team are currently optimising the shapes and geometrical arrangements of the nanoparticles to achieve optimal rates of photochemical rates. Through this optimisation process they are addressing key fundamental questions, which will provide guidance in the development of processes for creating complex hydrocarbons from carbon dioxide.
You can read more about this project in The Dark Side of Plasmonics, published in Nano Letters.
Small fibres solving big problems

The design of the needless electrospinning machine created at Deakin University
February 2014
The creation of fibrous materials is proving advantageous in a range of applications, from apparel fabrics and upholsteries to advanced composites, filters and biomedical scaffolds. The diameter of the fibres in these fabrics plays an important role in determining their functions and applications.
The smaller the fibres, the more potential they have in many fields. For example, the efficiency of fibrous filters increases with reduced fibre diameter and tissue scaffolds made of smaller fibres can provide better cell growth environment.
However, due to the difficulties associated with producing nanofibres on a large scale, commercial fibres typically tend to fall within the micron range, rather than the nano range.
Several techniques have been developed to increase the large scale production of nanofibres, the most versatile of which is electrospinning. Electrospinning is distinct from other nanofibre production techniques in its versatility to process different polymers, ability to control fibre diameter, morphology, orientation, component and fibrous structure.
However, the key challenge in electrospinning is low outputs due to the use of needle nozzles to produce the nanofibres. Prof Tong Lin, Dr Haitao Niu and Dr Jian Fang at Deakin University are aiming to solve this critical problem by using needle-free fibre generators to mass-produce quality nanofibres.
Through funding support from ANFF, Prof Lin, Dr Niu and Dr Fang have been able to successfully construct coil-based, needleless electrospinning machines, which have much higher fibre productivity than needle-based electrospinning. This manufacturing platform represents a breakthrough in production of quality nanofibres as it allows production of nanofibres on a large scale and development of various nanofibre products. Nanofibre webs can be produced continuously with a width up to 2 metres.
Some possible applications for these nanofibes include face masks and respirators for filtration of pollution from airborne particles. Microfibre masks are not adequately able to protect people from inhaling particles smaller than 2.5 microns, a serious issue in many industrial environments and countries. By adding a thin layer of nanofibre web into face masks, the filtration efficiency can be increased dramatically to block almost all tiny particles, thus providing best possible protection.
Another application of electrospun nanofibres is in Lithium ion batteries as separators. Battery separators, which are sheets of porous nanoconducting material, prevent short circuiting of the batteries by preventing the positive and negative plates from bending and touching. When made of electrospun nanofibres, they can extend the battery lifetime by 25%, without sacrificing electrical performance.
Furthermore, nanofibres electrospun from piezoelectric polymers show excellent ability to convert mechanical energies into electric power. The researchers found that needleless electrospinning can produce these power-generating nanofibres with much higher productivity than conventional needle electrospinning.
This research provides opportunities for local industry to manufacture nanofibres and develop nanofibre products. This would strengthen the technical ability of Australian local industry in producing nanofibre products, such as highly efficient air filter, face masks, Lithium ion battery separators and noise absorbers.
The next stage of this research will be focused on the development of these nanofibre-based products in collaboration with industry. Efforts will also be made to refine the performance of the electrospinning machines.
Micro sensors to monitor blood pressure
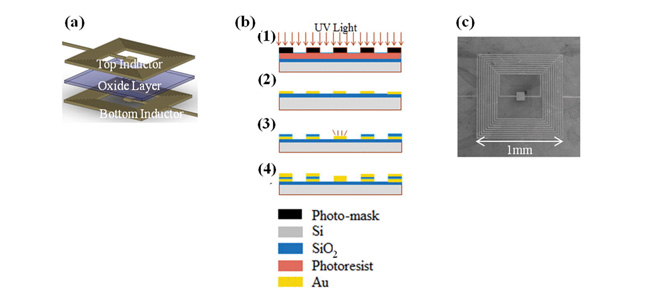
(a) An exploded view of the double layer planar micro inductors, (b) A summary of the fabrication steps: (1) Oxide deposition and UV Lithography, (2) E-beam evaporation and lift-off. (3) SiO2 deposition and etching. (4) Steps 1–2 repeated for the second inductor layer, (c) A scanning electron micrograph of the resulting coil.
October 2013
Hypertension, if left untreated, can lead to a number of health problems including strokes, heart failure and kidney failure. Regular monitoring of blood pressure is currently inefficient and is not performed as regularly as necessary to monitor and mitigate these serious health concerns.
Led by Dr. Tuncay Alan, researchers from Monash University are developing a highly sensitive, implantable blood pressure sensor. These micro-sensors could be implanted into patients suffering high blood pressure to better monitor levels. This would allow continuous and routine blood pressure measurement and readings would then be communicated wirelessly to a hip-held alert unit. The results could then lead to earlier diagnoses of related diseases, complications and more efficient treatment.
The devices being investigated by Dr Tuncay Alan and Associate Professor Neild in the Laboratory for Microsystems, are inductor sensors which are far more sensitive when downscaled than other sensors which have previously been researched. The team have developed a model which uses two planar spirals separated by an insulating layer, and have fabricated double layered microplanar coils at MCN. The opposing magnetic fields between pairs of these coils create a high sensitivity to relative displacement which can be linked to pressure. Results so far show that device dimensions could be shrunk for further improvements on resolution. Overall results indicate that inductive sensing has a good potential for micro scale sensing applications. Collaborators are currently investigating the circuitry necessary for the sensor and the fabrication of a complete system.
You can read more about this project in Nanoscale displacement sensing using microfabricated variable inductance planar coils, published in Applied Physics Letters.
Microflow batteries the future for electric cars
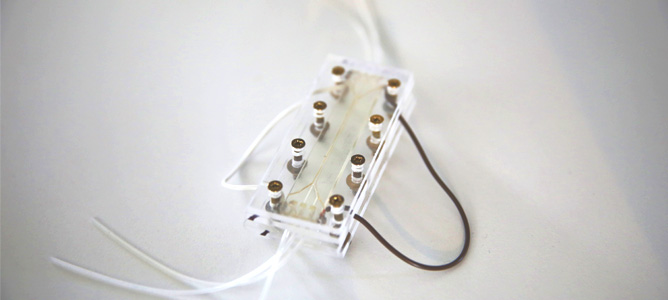
A micro-flow battery developed by Eden Research and Development
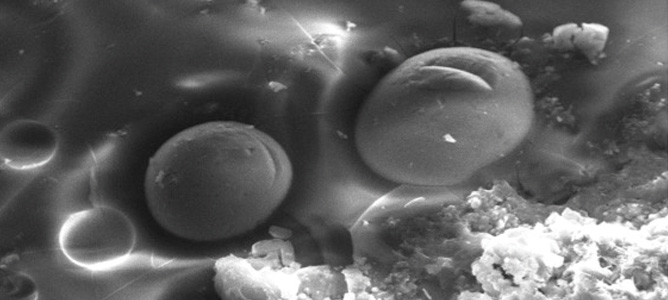
An Edenglass nanoparticle emerging from metallic oxide glass
July 2013
Eden Research and Development, an unlisted public company, has taken residency at MCN to develop a number of energy-related inventions. The first device built at MCN is a micro-battery using self-assembling nanocapacitors derived from proprietary metallic oxide glass with potential application as an electric car battery (or other energy storage applications) due to its size, safety and low cost profile.
The Eden micro-battery is believed to be the world’s first microfluidics battery using a miniaturised electrolyte (vanadium) flow battery design. In a flow battery, the electrolytes are charged and discharged in a cycle that is repeated thousands of times. The benefit of flow batteries is that they can be discharged down to zero charge at every cycle without suffering damage.
The overall construction of the chip was made on the MCN Eden 260 3D printer using a special transparent epoxy acrylate resin. The micro-battery uses a third electrolyte stream containing nanocapacitors instead of an ion permeable membrane that is normally used in vanadium flow batteries, which results in a substantial increase in both power and energy density.
An advantage of this micro-flow battery in an electric car will be that it can be recharged from the recovered energy of the moving car, minimising the recharge time required for the vehicle. The method used to collect and store this energy comes from a vitreous catalyst constructed from nanoparticles. These particles are nano sized capacitors, which are understood to attract charged ions, store them in its surface skin and be available on demand. The flow of ions in the cycles triggers the nano capacitors to release the ions and contribute to the current.
Eden’s Technical Director, Dr. Ross Mack said “We are very grateful to MCN for their assistance to date. The tools and instruments available at MCN are excellent, the staff are highly qualified and the Victorian Government is to be congratulated for having the vision to create the centre.”
For more information, visit www.edenbdm.com
NB: The material for this case study has been provided by Eden Research and Development. Although care has been taken to ensure the accuracy and completeness of the information that is provided, the MCN assumes no responsibility in relation to the interpretation or use of this information.
Harvesting sunlight to fuel the modern world
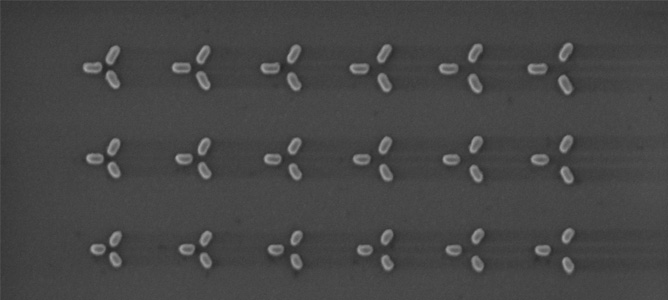
A Scanning Electron Microscope (SEM) image of a set of nanoparticles designed for capturing light. The basic design consists of an isosceles triangle of nanorods. Each nanorod is made of gold. By changing the sizes and separation among the nanorods, we can control which part of the solar spectrum get trapped by the nanoparticle trimer.
March 2013
Harvesting energy from light sources, including the sun, could provide a significant percentage of the world’s energy needs over the next century. In order to meet this critical demand, it is crucial to devise ways to transform light energy into chemical fuels, not just electricity. The aim of this project is to create new nanostructures that directly couple light energy into chemical reactions. In this study, researchers from CSIRO and the University of Melbourne, led by MCN Technolgy Fellow Dr. Daniel Gomez, performed the first step in this process - capturing and storing light energy with a system of nanoparticles.
The team created a system of nanoparticles that captures a significant amount of light which is able to store this energy for longer than most traditional systems. This feat is achieved by suppressing “re-radiation of light” by using metal nanoparticles that do not efficiently radiate light. The basic design consists of three metallic nanorods arranged in the shape of an isosceles triangle.
The metal nanoparticles were firstly designed at the CSIRO and The University of Melbourne using a sophisticated but simple theoretical model. The design was later transformed into real nanoparticles at MCN and was later put into action by testing their optical properties at the Optics laboratories at the University of Melbourne.
The nanoparticles were created using the electron beam lithography tool in the MCN cleanroom in addition to high resolution imaging equipment (scanning electron microscope), optical characterisation equipment (spectroscopic ellipsometer) and advanced metal processing tools (electron beam evaporator).
The most significant aspect of this project is that the team have achieved light trapping at the nanoscale by design. Starting with a highly sophisticated theoretical model of nanoparticles, they were able to create a structure that was able to interact with light (of particular wavelength and polarisation) and trap it more efficiently than most common systems. No single publication to date comprises a set of experimental and theoretical results as consistent and homogenous as the one presented by the group on the subject of ‘dark plasmonic modes.’ Due to the experimental precision, the excellent qualitative agreement between experimental data and the theoretical model and the large parameter space investigated, their study is a strong first step in the greater goal of creating solar fuels.
This study leads the way in a succession required for demonstrating solar-to-chemical energy conversion. While the general public often associates solar energy to the generation of electricity, sunlight can also be used for driving important chemical reactions such as the production of solar fuels. Some of these fuels include hydrogen gas, whose only product after combustion is water (not even a small trace of CO2, thus a fully carbon emission free technology). The outcomes of this grand challenge, namely using nanotechnology for solar-to-chemical energy conversion, will lead to low emissions, renewable energy sources and to a future Australian nanomaterial manufacturing industry.
After capturing light, the next step is to devise ways to do useful work with the energy trapped from it. The team at MCN will incorporate the nanoparticle designs into advanced architectures to demonstrate key chemical reactions driven entirely by sunlight.
Cancer treatment to be delivered to lungs
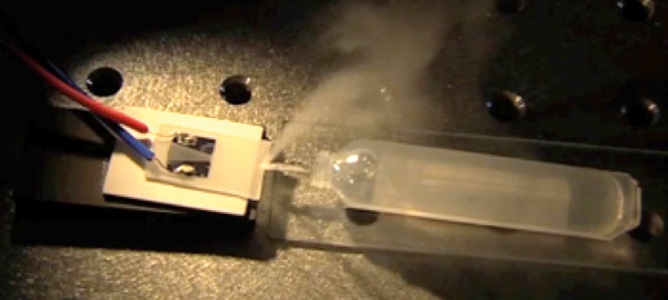
Surface acoustic waves propagate along the surface of the nanofabricated Respire® device, leading to the atomisation of drug solutions into a fine mist suitable for inhalation.
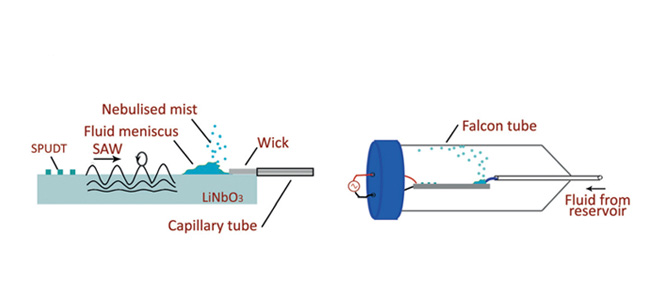
A schematic of the SAW nebulisation mechanism which propagates along the substrate and leaks energy into the liquid film to nebulise the meniscus. Collection of the aerosolised DNA or antibodies is accomplished by placing the device within a conical tube.
March 2013
In treating diseases such as lung cancer, tuberculosis, cystic fybrosis and asthma, a large range of intravenous drugs are directed at the lungs but inefficiently delivered. This has led to significant research into devices that can deliver drugs to the lungs effectively and efficiently. A device under investigation for the generation and delivery of aerosolised drugs is the Respire® system.
At present, many large drugs directed at the lung, including antibodies, are injected intravenously because of the lack of effective inhalation devices. The Respire® inhalation system would enable a portable way of delivering large macromolecular drugs straight to the lungs where they are needed, creating a high local concentration of active drug in the lung to improve patient outcomes.
MCN Technology Fellow, Christina Cortez Jugo from Monash University and colleagues from RMIT University are investigating the formation of inhalable droplets of clinically significant drugs including monoclonal antibodies for cancer therapy and asthma. Promising results show that these fragile drugs remain stable and active after atomisation, paving the way for further testing of their efficacy in treating disease.
The device, developed by Professors James Friend and Leslie Yeo, was fabricated at MCN using standard photolithography techniques. The technology is based on surface acoustic wave (SAW) atomisation. When power is supplied to the miniature device, waves akin to nano-earthquakes travel along the device causing the destabilisation and atomisation of liquid droplets in its path. A fine mist suitable for inhalation is formed.
The group atomised a solution of monoclonal antibodies targeted against the epidermal growth factor receptor, which is over-expressed in lung cancer. The stability, immunoactivity and function of the atomised antibodies were characterised using gel electrophoresis, confocal microscopy and flow cytometry. The results indicate that the Respire® system provides a feasible means of delivering active antibodies as a fine inhalable mist to the lung. Collaboration with Dr. Manuel Ferreira at the Queensland Institute for Medical Research is in place to test the device for the biodistribution and efficacy of the atomised antibodies.
In collaboration with Dr. Manuel Ferreira, the group will be undertaking biodistribution and efficacy studies of inhaled antibody formulations in mice. In addition to monoclonal antibodies, the Respire® system is also being investigated for the inhalable delivery of nanomedicinal formulations and nucleic acid drugs, including small interfering RNA or siRNA for gene silencing applications. Access to the capabilities and expertise at MCN will continue to be important to facilitate the ongoing and future work in this project.
You can read more about this project in Enabling practical surface acoustic wave nebuliser drug delivery via amplitude modulation, published in Lab on a Chip.
A pain-free solution to vaccines
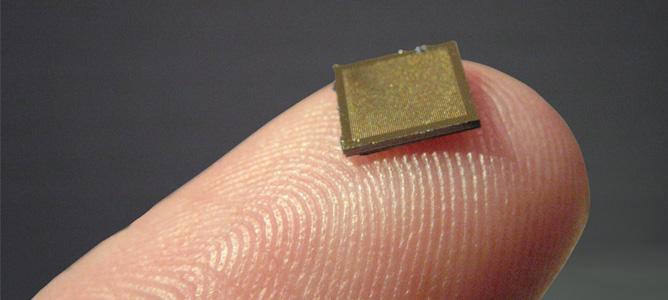
A NanopatchTM placed on a finger to demonstrate its size and application
October 2013
Vaccinations, though vital in preventing the spread of disease in the modern world, have several major drawbacks which limit their effectiveness and uptake in developing countries.
Professor Mark Kendall from the Australian Institute of Bioengineering and Nanotechnology, has spent the last ten years developing the NanopatchTM - a needle free solution to many of the problems facing vaccine use. A tiny square of microneedles coated in vaccine, the Nanopatch is simply placed on the skin and the vaccine dispersed into the immune system just below the skin.
Vaxxas Pty. Ltd., which was established to develop the NanopatchTM, engaged with MCN to increase the outputs of their trial patches. By providing Vaxxas with access to state-of-the-art development equipment and expertise, MCN has accelerated and improved the production of the NanopatchTM.
The NanopatchTM is manufactured using mono-crystalline silicon wafers which are subjected to deep-reactive ion etching (DRIE), the same proccess that is commonly used to fabricate components of smart phones and other microtechnology devices.
MCN has helped to fine tune the NanopatchTM production process so that the layers deposited on these wafers achieves better uniformity, increasing the output from each wafer. Furthermore, MCN has allowed Vaxxas to move from four inch wafers to six inch wafers, once again increasing the yield. MCN also hosts two DRIE systems which enables them to double their final outputs by processing wafers in parallel.
Eliminating the pain of traditional injections is just one small advantage of the NanopatchTM. As the vaccine is dry coated onto the patch, the need for refrigeration is eliminated. This vastly increases its stability and reduces cost barriers for transporting vaccines to where they are needed most in third world countries. The NanopatchTM has the capacity to transform the world’s disease landscape, and to stamp out illnesses in third world countries that have long been preventable in the developed world.
NB: The material for this case study has been provided by Vaxxas Pty. Ltd. Although care has been taken to ensure the accuracy and completeness of the information that is provided, the MCN assumes no responsibility in relation to the interpretation or use of this information.
More information can be found at www.vaxxas.com.au
Microchips to mimic living cells

A microchip that can generate microdroplets for protein and nanoparticle synthesis.
July 2013
Together with MCN, Technology Fellow Yonggang Zhu and his team from CSIRO have successfully developed a microchip that mimics a living cell by housing millions of tiny water droplets which synthesise enzyme molecules. This is a critical step towards fast-tracking low cost protein development.
They have successfully developed a microchip that can produce millions of tiny water droplets inside itself. These droplets contain necessary materials like those in a living cell, while enzyme molecules are synthesised in each droplet. The unprecedented speed of synthesis can potentially solve some of the bottle-neck problems in protein evolution.
The team has studied the physics of fluid flow at microchannels and designed a microchip that can flow two immiscible flows i.e. water and oil, for generating monodispersed water-in-oil microdroplets. The microchip was fabricated at MCN using polymeric materials instead of silicon. The plastic microchip has the function of sequentially generating microdroplets for compartmentalising biological reagents, performing in-drop in vitro transcription and translation reactions, fusing droplets for on-chip enzyme assays and detecting in-droplet biochemical reaction products.
The microchip format allows the generation of millions of enzyme assay droplets per day, which is an unprecedentedly high throughput none of the currently commercially available equipment can match. The progress Dr. Zhu’s team has made in microchip-based enzyme synthesis and screening is significant. Since tiny amounts of sample solution is required and a high throughput can be achieved, this could potentially allow researchers to screen and select libraries of massive numbers (from millions to trillions) of candidate gene/protein variants, from either natural sources or synthetic ‘in vitro evolution’ experiments, a critical step to fast track the protein development with low cost.
The technology could potentially allow biologists to develop new proteins (e.g. enzymes) within days instead of years and with a cost of hundreds of dollars instead of millions and billions of dollars. This will benefit many areas in biotechnology, medicine and environment.
You can read more about this work in Enzyme synthesis and activity assay in microfluidic droplets on a chip, published in Engineering in Life Sciences.