Next generation pathogen detection with lab-on-a-chip platforms
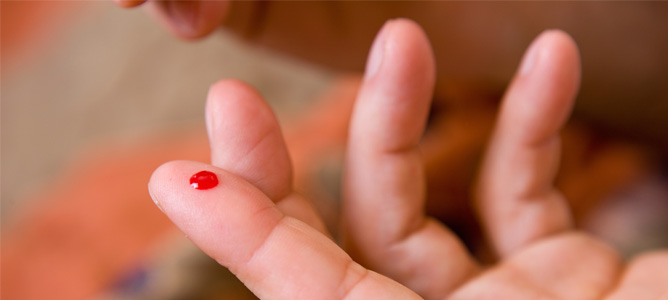
Taking a sample could be as simple as a fingerprick of blood, as is common in blood sugar monitoring.
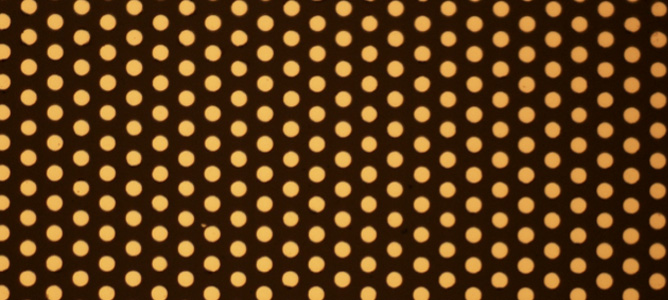
The metal microdiscs sputtered on a glass slide at MCN. The posts are 5 µm in diameter and 300 nm high.
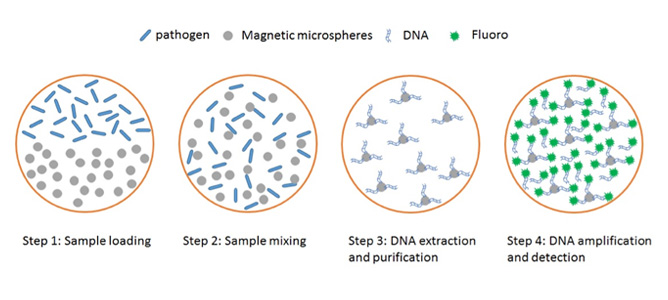
The schematic drawing of the pathogen detection: DNA extraction, purification, amplification and detection.
March 2015
Detection of pathogens is of critical importance for disease diagnosis, food safety control, water quality monitoring and homeland security. Traditional detection methods, such as colony counting following cell culture, polymerase chain reaction (PCR) and enzyme immunoassay, have been extensively employed to pathogen detection. But they require special laboratories, trained professionals and hours, if not days, to achieve the required analysis.
The portable lab on a chip platform developed in this project enables the detection of multiple pathogens within 30 minutes, with only a tiny sample, such as a drop of blood or saliva. The detection process is as easy as blood sugar monitoring used daily by diabetics: drop the sample on a disposable microchip, measuring just 5cm x 7cm x 0.4 cm, insert the chip into the reader and read the results after half an hour. It is fully automatic and no longer relies on sophisticated equipment and trained professionals. Furthermore, it not only greatly reduces the complexity and detection time but dramatically increases detection sensitivity. It holds great potential to benefit the medical clinics, food industries, as well as departments of environmental monitoring and homeland security.
Led by Dr. Yonggang Zhu, CSIRO researchers from multiple disciplines have been collaborating with industry partners to develop a lab-on-a-chip platform with three key capabilities including automatic liquid handling, sample processing and individual bead trapping and imaging. The liquid handling system is applied to load the samples (such as blood, saliva, urine, etc.) and reagents are placed into a microwell for further processing. Then magnetic microbeads and an electromagnetic/acoustic system are applied to mix and capture the analyte. Finally, the microbeads coupled with analyte are individually captured and imaged by fluorescence microscopy or Surface Enhanced Raman Spectroscopy (SERS).
The core component of this platform is the microfluidic chip with microchannels, a microwell and metal microstructures for the sample processing and detection. It is fabricated using the photolithography facilities and sputtering tools at MCN.
Proof of concept studies have shown successful detection of DNA from E. Coli (bacteria) using this lab on a chip platform. DNA was lysed from whole E. Coli cells and purified on the chip within 15 minutes. Then the small number of DNA molecules were amplified in situ for a further 15 minutes. Following the amplification, concentrated DNA molecules weres able to be detected using fluorescence microscopy.
Future work will focus on the optimisation of the platform in terms of hardware, detection methods and software to further shorten the detection time, reduce the detection limit (therefore detecting fewer pathogens in a given volume) and increase its ability in detecting multiple pathogens.
Twisting light for faster internet
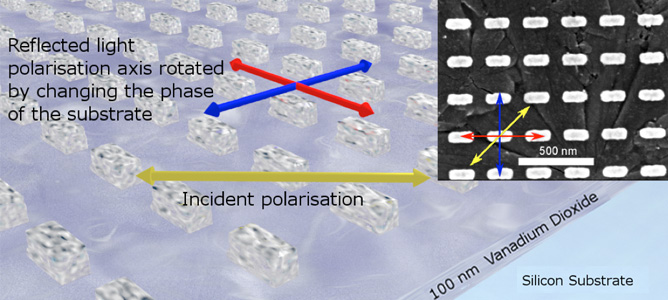
Diagram of the polarisation rotation device. Inset: SEM image of silver nanorods on vanadium dioxide-coated silicon substrate.
March 2015
With the advent of fibre optic networks the bandwidth of the internet increased dramatically. The bottlenecks to next-generation internet speeds are now the points at which the light carrying the signal is switched to an electronic signal.
By replacing current electronic switches with optical equivalents, these bottlenecks can be removed increasing throughput while reducing power consumption, waste, heat and maintenance costs.
A collaborative project between researchers at the University of Melbourne, MCN, CSIRO and Vanderbilt University has created a device which enables the user to rotate the reflected linear polarisation axis of light. Used in conjunction with a pair of linear polarisers this allows a passive routing of the reflected signal. The working is a phenomenon known as a localised surface plasmon (LSP), which is caused by resonant oscillations of the free electrons confined to a metallic structure smaller than the wavelength of light. An array of rods, each displaying two of these LSPs parallel to their two axes, permits altering the polarisation state of that light. By carefully setting the dimensions of the rods, it is then possible to use these LSPs in conjunction with a phase change material to rotate the reflected light’s polarisation axis. Dramatically increased optical switching is relevant for a range of optical devices from microprocessors and electronics to sensing and telecommunications.
Electron beam lithography and evaporation of ultra-thin silver films were used at MCN to create optical antennas on vanadium dioxide, a phase change material deposited by collaborators at Vanderbilt University. Atomic force microscopy and scanning electron microscopy was then used to characterise the vanadium dioxide film and image the antenna arrays. The facilities at the MCN enabled the refinement of the fabrication process and creation of the actual device.
This project has shown how a simple structure can twist the polarisation of reflected light. In principle this could be further developed such that its operation is ultra-fast. While the crystalline phase of vanadium dioxide was transitioned thermally, it has the potential to be switched optically on a sub-picosecond timescale, enabling switching orders of magnitude faster than current technology.
The device has been shown to work in the visible part of the spectrum, however moving the design to telecommunications wavelengths (near infrared, 1550 nm) will facilitate device longevity by enabling the use of gold rather than silver, as well as simple integration into current fibre optic networks. Long-term plans aim to implement a simple self-switching design using amplitude modulation of the incident signal.
Artificial leaves enhance light-to-chemical energy conversion
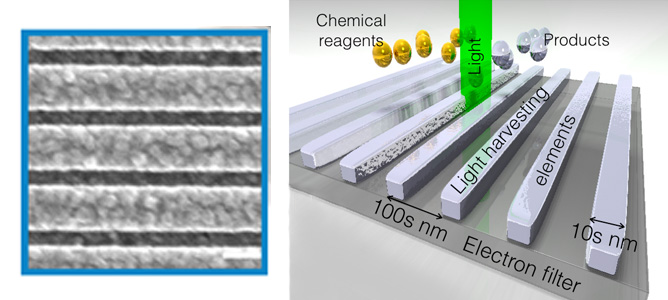
Left: SEM image of the top view of a fabricated artificial leaf (light harvesting structures), where the white bar corresponds to a length of 200 nm. Right: Diagram illustrating the artificial leaves and the size scales involved. Credit: D. Gomez
March 2015
Modern society is, to a very large extent, built on the products of the organic chemical industry. On a daily basis, these products are used for making pharmaceuticals, plastics, health and cosmetics products and for several items in the housing, transportation and telecommunication industries.
The chemical industry relies heavily on fossil fuel derived energy for carrying out these chemical processes. A more sustainable and desirable prospect is to harvest sunlight for doing chemistry and thus transform this industry into a solar chemical manufacturing industry.
This project aims to create novel ways to harvest solar energy for driving chemical reactions instead of generating electrical power. To this end, a team of researchers from CSIRO and the National Institute for Materials Scienc design in Japan, have fabricated nano-sized structures that are capable of harvesting light and converting it into chemical potential energy.
They have used the high-resolution electron beam lithography tool at MCN to create structures capable of efficiently harvesting light for driving chemical transformations, with some assessment of their performance carried out by collaborators in Japan.The artifical leaves, or light harvesting devices, are made out of a light harvesting component in direct contact with an electron filter. Each light harvesting element is made of Aluminium wires with a cross section of tens of nanometers. The spacing between the light harvesting elements is a critical parameter, with its magnitude is in the hundreds of nanometers which is controlled with a 10nm resolution.
Through this project they have demonstrated up to two orders of magnitude improvement in the rate of the chemical reaction of a test model using their light-harvesting system. They have achieved this by using a very simple configuration of nanostructures and anticipate that more spectacular light-to-chemical energy transformations can be achieved with more sophisticated nanoparticle deigns.
The team are currently creating more sophisticated nanoparticle systems that are capable of absorbing nearly 100% of the incident light across the visible spectrum. This will translate into much higher light-to-chemical energy transformation efficiencies and the team envisage that these light-harvesting technologies could be used for developing a sustainable and solar driven fine chemicals manufacturing industry in the not too distant future.
Nano-flowers for biosensing
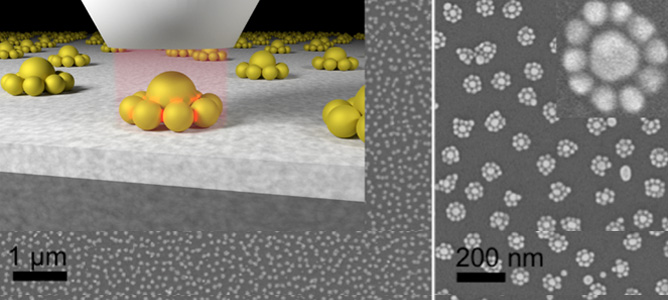
Schematic representation of the nano flowers focusing incoming light in the gaps. Overview SEM image on the left and zoomed-in views of the structures on the right showing the nano flower arrays. Credits: Soon Hock Ng, Yuanhui Zheng.
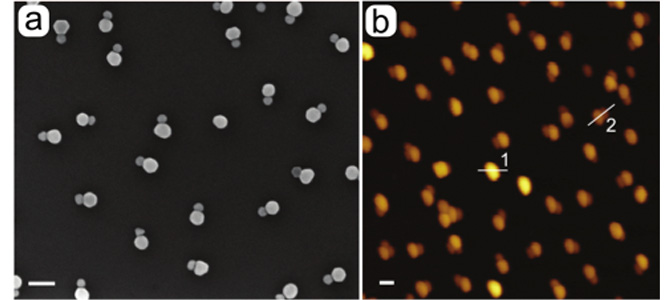
Pairwise assembled nanoparticles imaged with SEM (a) and AFM (b).
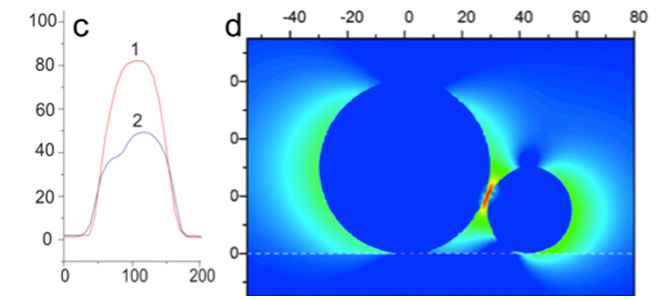
Vertically stacked particles can be found in the AFM images by using line scans in the AFM images (c). The yield of dimers with the here developed protocol is as high as 85%. (d) shows a calculation of the field focusing in the gap of such a dimer (red indicates a high intensity).
March 2015
Gold nanostructures separated by a small gaps (1/10,000 the thickness of a human hair) show a strong focusing effect of the electric field into this gap when exposed to light. Assembly of nanoparticles in flower structures with multiple of such gaps, have been developed. Using a spectroscopic technique known as Surface Enhanced Raman spectroscopy (SERS), these structures have the potential to detect minute quantities of molecules used in explosives or cancer markers in blood.
A team of researchers led by MCN Technology Fellow, Associate Professor Udo Bach from CSIRO have attached DNA onto gold nanoparticles to give them a negative charge. Small glass surfaces were given a positive charge, after which the glass is placed into the gold particle solution. This enables a regular distribution of particles on the surface. DNA requires two different parts to form a double helix - the DNA on the surface particles contains one such part, while a solution of smaller particles has the other part of the DNA attached to it. When the glass is placed into the solution with the smaller particles, the DNA comes together to form a double helix and connects the smaller particles to the larger ones, leading to the flower structure seen in the image above.
The assembly of these flowers was carried out in the MCN biochemistry laboratory, while the progress and results have been monitored using MCN’s UV-Vis, SEM and AFM tools.
In comparison to commercially available SERS sensor chips, the flower structures has proved to be superior in signal intensity and reproducibility. This is particularly interesting, as a new and simpler fabrication method for these complex structures has been used in this study.
Besides the improvements in molecular sensing, the techniques developed in this project may be used to control the assembly of nanoparticles of various materials and therefore applications in catalysis are envisaged, for example. This is particularly attractive in times where renewable energies and processes like water splitting are of high interest.
Future stages of the project aim to continue in the same vein, as electrostatic forces can be used to assemble nanoparticles in pairs. Similar hotspots as in the flower structures can be produced in an even simpler way, while the effect of the size and the material of the nanoparticles on the performance may help to unlock new applications for such structures.
ALD could help prolongue battery life and improve reliability
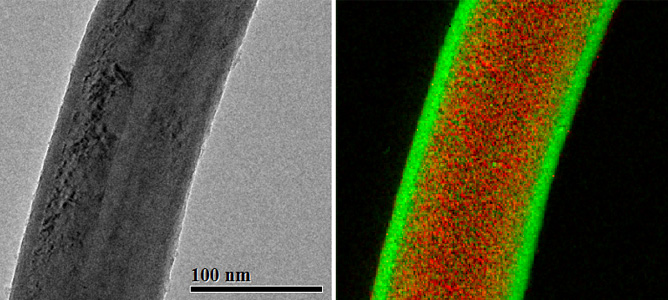
Left: A boron nitride nanotube coated with a 10 nm conformal layer of aluminium oxide (a bright-field TEM image); Right: energy-filtered image with elemental contrast (green – oxygen, red - boron).
November 2014
Improving the charge and life-span of batteries in phones, laptops and household technologies has the potential to improve not only efficiency and flexibility of these technologies, but also minimise the amount of toxic landfill created every year as a result of disused batteries.
MCN Technology Fellow, Dr. Alexey Glushenkov has been working on electrode materials for lithium ion and sodium ion batteries as well as electrochemical supercapacitors. A recent trial as part of this project saw a conformal deposition of 10nm of aluminium oxide onto boron nitride nanotubes by Atomic Layer Deposition (ALD). A normally challenging structure to coat uniformly, the nanotubes were coated by a homogeneous, 10nm thick layer of aluminium oxide using MCN’s ALD Savannah S100 instrument. The ALD techniques available at MCN are able to achieve highly conformal, continuous, pin-hole free depositions on complex surfaces and porous structures.
Dr. Glushenkov is aiming to use a similar technique to provide coatings for electrodes in batteries to provide an artificial surface layer to separate electrodes from direct contact with electrolytes in batteries. Such a surface layer is capable of ionic conduction and does not interfere with battery operation, while it works to reduce unwanted side effects caused by the contact of electrodes with electrolytes and result in better cyclic stability and reliability of batteries.
This project holds great potential for revolutionising the portable electronics industry and the way we interact with our devices.
Fighting resistance to antibiotics
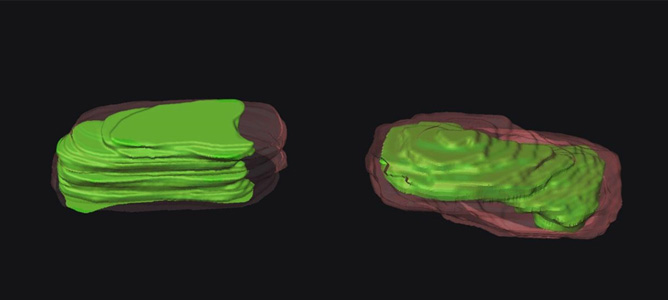
Reconstructed 3D models of single, untreated bacteria (left) and treated with 2 mg/L polymyxin B (right).
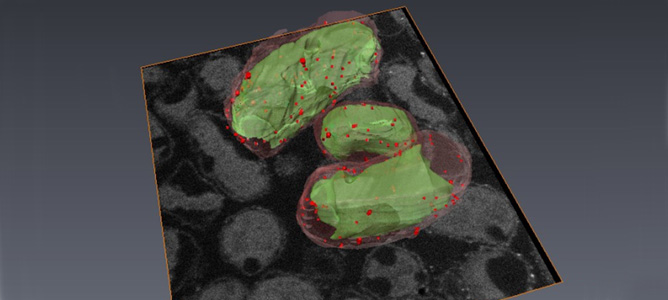
Three bacterial cells reconstructed using FIB-SEM tomography.
November 2014
As resistance to antibiotics becomes increasingly common, it is more important than ever to understand the mechanism by which antibiotics work on bacterial cells, and to develop new antibiotics which can be used against ‘super bugs.’
In order to understand the process by which potential combinations of antibiotics kill multi-drug resistant bacterial cells, a joint force from Monash Engineering (Mr. Boyin Liu and Dr. Jing Fu) and Monash Pharmaceutical Sciences (Professor Jian Li and Dr. Tony Velkov) together with the MCN, the Australian Synchrotron and the University of Queensland have been working on novel imaging approaches to assess the cellular responses of bacterial cells to the treatment of antibiotics, including the last-resort polymyxins.
The team has used the Focused Ion Beam (FIB) tool at MCN to mill away 25nm slices of a cell of resistant bacterial isolate (Klebsiella Pneumoniae) recently discovered in Queensland. After the removal of each slice, high-resolution scanning electron microscope images were taken and then reconstructed into a 3D model of a whole bacterial cell to reveal the effect of the antibiotic in different cellular regions.
The 3D models of both treated and untreated cells were reconstructed and compared. Their finding confirmed the invasion of polymyxin B on the cell envelope and the subsequent depletion of cytoplasmic materials. The results provided clear evidence for using rational antibiotic combinations to combat bacterial ‘superbugs.’
In the ongoing research, FIB is also being used to slice the bacterial cell to expose the interior surface, after which Atomic Force Microscopy (AFM) is employed to probe the intracellular changes and measure their mechanical properties. The investigators are also employing a single-molecule AFM tip functionalisation technique, Synchrotron imaging and secondary ion mass spectrometry to identify the chemical signatures due to antibiotic treatment. Their research is funded by the Australian NHMRC and the US National Institutes of Health (NIH).
You can read more about this project in In situ probing the interior of single bacterial cells at nanometer scale, published in IOP Science in September this year.
High resolution x-ray imaging possible for delicate biological samples

Top view of the 50nm resolution zone plate with the 30µm cross section.

52o tilted view of the FIB cross-cut of the outer zones

Top view of the 38nm resolution zone plate
April 2014
High-resolution x-ray imaging is required to investigate processes in-situ or in-vivo samples or when it is particularly important that the sample is not damaged. This is possible due to the high penetration depth of the x-rays. Fresnel zone plates are optical elements that are capable of focusing x-rays with high efficiencies, allowing for nanometer size beams to deeply penetrate the imaging subjects.
A collaborative project between the MCN, La Trobe University and the Australian Synchrotron aims to fabricate high-resolution Fresnel zone plates and test their performance using synchrotron light.
As a starting point, a theoretical model was used in order to predict the highest achievable performance of zone plates for a given set of parameters. Subsequently, a fabrication protocol was chosen to produce these optical elements. The protocol involved testing a wide range of techniques including silicon wafer processing, photolithography, reactive ion etching, wet etching, electron-beam evaporation, electron-beam lithography, electro-deposition, and focused ion beam. The successful prototypes were tested at the Australian Synchrotron and their performance was measured against the theoretical predictions.
By utilising state-of-the-art instrumentation at the MCN to test theoretical predictions, the researchers have been able to create zone plates which exhibit properties that are close to the theoretical calculations. These zone plates are able to focus large diameter x-ray beams into nanometer-size spots which have high brilliance. This is important as it delivers not only high-resolution imaging capability, but it also minimises the exposure times which in turn maximises the data output capacity.
Furthermore, current prototypes incorporate in-built elements that act as x-ray beam stops, eliminating the need for additional stages usually required to mount external beam stops. This also limits the errors associated with the alignment process.
The benefits of using such optical elements are enormous as this is currently the only way to focus x-rays to nanometer-size beams with high efficiencies. This is paramount when high-resolution x-ray imaging is required to nondestructively investigate a diverse range of scientific problems.
The next stage of this project will be to produce prototypes on demand for the Australian Synchrotron scientific community to benefit diverse range of X-ray imaging techniques.
Electronic skins – the future for medical devices
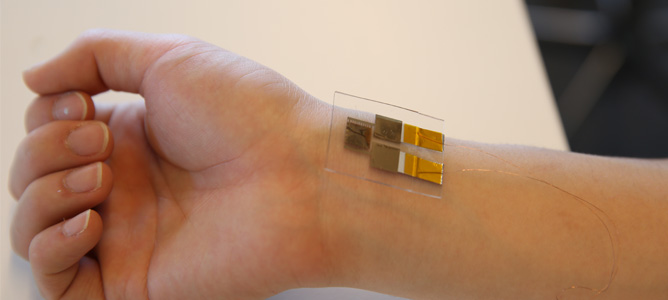
A sample of the wearable sensor.
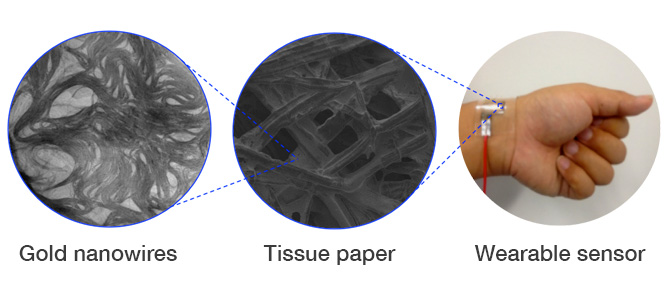
The impregnation of tissue papers by ultrathin gold nanowire inks lead to highly-sensitive and wearable pressure sensors.
July 2014
Technologies are increasingly becoming smaller, lighter and smarter, and now they are becoming flexible and wearable too. Current rigid circuit board technologies are limited in their applications, particularly with regards to medical devices and bionics. MCN Technology Fellow, Professor Wenlong Cheng from Monash University, has been leading a team in the development of stretchable, elastomeric conductors for use in pressure sensing.
Electronics such as these which are soft, flexible, stretchable and wearable are enabling applications that are impossible to achieve with circuit board technologies. The focus of this project is the creation of pressure sensors which enable portable pulse and heart rate monitoring, as well as body movement sensing.
These sensors are not achievable with traditional organic or inorganic materials or conventional manufacturing methods. Utilising the facilities at MCN, Professor Cheng’s team have developed a simple yet efficient and low-cost nanotechnological approach to integrating ultrathin gold nanowires into tissue paper to create an ultra-flexible sensor. This can then be sandwiched between thin layers of PDMS and attached to wiring to create a wearable sensor that can provide readings of blood pressure, heart rate and movement. Such sensors are so sensitive that tiny forces from blood pulses and acoustic vibrational forces can be accurately detected.
This development of soft electronics holds huge potential for portable health monitoring devices. Professor Cheng will be looking at the commercial development of this project next.
Professor Cheng can be heard discussing these sensors on the BBC radio program, Click. The interview starts approximately 6 minutes into the show.
You can read more about this project in the Nature Communications Paper, A wearable and highly sensitive pressure sensor with ultrathin gold nano wires, published in February 2014.
Nanoscale optical circuits for light-speed information processing

An example of a nanoscale optical circuit that measures optical phase difference. a) A graphic of the circuit which consists of three gold nanorods supporting localised surface plasmon resonances. One of the nanorods outputs an optical signal proportional to the difference between the two inputs. b) An SEM image of the resist pattern on a gold film prior to etching.

c) A microscope image of a circuit when the optical phase difference across the inputs is zero. The circuit cannot be seen because there is no output for zero phase difference. d) A microscope image of a single circuit when the phase difference is 40o. The circuit is too small to resolve but the light output from it is easily observed.

Two examples of nano-plasmonic circuits based on evanescent coupling between localised surface plasmons that show the circuit diagrams and their representations as metal nanorods.
April 2014
Modern communications networks that support the internet rely on light waves to carry information. In these systems, digital signals are imposed on the light beams, which travel long distances on optical fibres.
Currently these systems rely on electronic devices to encode and decode the information. While electronics can be integrated into high-density circuits, the limited speed at which these circuits function limits the amount of data that can be encoded on the light beam. Conversely, optical circuits with much faster speeds can be created using optical waveguides integrated into silicon chips, however they cannot achieve the same density as electrical circuits.
Led by MCN Technology Fellow Dr. Tim Davis, researchers from CSIRO have been utilising the MCN facilities to develop nano-scale optical circuits which operate at exceptionally high speeds and which can be integrated into high-density circuits. These ultimately demonstrate optical information processing.
The circuits are based on configurations of gold nanorods that support localised surface plasmon resonances – electric charge oscillations that are excited by light on metal surfaces. The team fabricated an optical circuit that was barely 200nm wide which measured the phase difference of an incident light wave across its inputs.
The circuit has many applications including the demodulation of optical signals with information encoded by phase shift keying. These nanoscale circuits operate extremely quickly and are able to respond to signals thousands of times faster than conventional electronic circuits, at a rate of up to 50,000 GHz.
To achieve these extremely fast nanoscale circuits, the team utilised the Electron Beam Lithography (EBL) tool at MCN. A glass wafer was first covered in a thin layer of gold about 30nm thick, after which an electron beam sensitive resist was coated over the gold. This was then exposed by the EBL. After the resist was developed, the circuits were imaged in the scanning electron microscope to check the integrity of the fabrication. The final step was to etch the gold film in the ion-etching instrument, which removes the gold unprotected by the resist. The result is a set of metal nano-rods that form the optical circuit.
While the optical circuits are too small to be imaged in an optical microscope, it is still possible to see the light scattered from them. The team was able to show that a single circuit could output an optical signal related to the phase difference of the light wave incident at its inputs. Incredibly, the entire device is about one quarter of the wavelength of light and probes the optical phase difference within a small fraction of a wavelength. This can be likened to a nano-scale voltmeter probing the electric field of the light wave.
The measurement of optical phase can be viewed as a form of optical signal processing. It is possible to show that optical circuits based on localised surface plasmons can perform a variety of linear mathematical operations between optical signals, all at the nano-scale. This project demonstrates that optical circuits of this type are feasible and there are a large number of possible circuits that can be designed, providing a means for processing information encoded on light beams.
The next steps in the project are to develop a range of other optical circuits and combine them. The team has already shown that arrays of optical circuits can be used to create a metamateria which enables the modulation and switching of one light beam by another. They will also be creating artificial materials based on these circuits that will be used for image processing.
You can read more about this project in Measuring subwavelength phase differences with a plasmonic circuit—an example of nanoscale optical signal processing, published in Optics Info Base.
Metal nanoparticles lead the way towards solar water decontamination
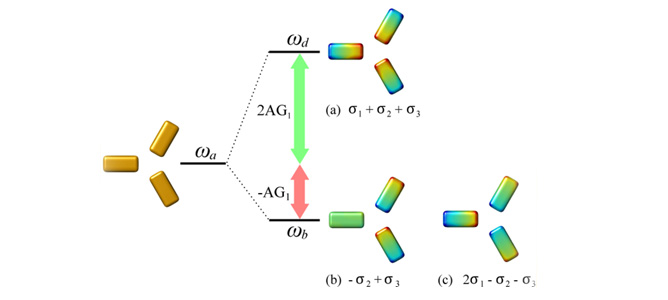
Three lowest-order modes of an interacting triangle of particles. Mode a) is the highest in energy of the three and is a mode with radial symmetry. Modes b) and c) are degenerate modes which are characterised by having a net dipole moment and perpendicular polarisations.
April 2014
It has long been known that solar energy can be used to create electric energy for heating and lighting. It can also be used however, to drive important chemical transformations such as the production of renewable chemical fuels by extracting hydrogen from water and hydrocarbons from carbon dioxide. Other uses include the sequestration of air pollutants such as greenhouse gases, low-cost water purification for developing countries, and the low carbon-footprint production of fine chemicals such as disinfectants and self–cleaning surfaces. There are countless other as yet unforeseen applications of solar to chemical energy transformations.
Existing approaches for harnessing solar energy for these applications are relatively inefficient and therefore not widespread. In order to improve the efficiency of solar-to-chemical energy conversion processes, it is important to maximise the light collection efficiency.
In this project, researchers from CSIRO led by MCN Technology Fellow Dr. Daniel Gomez, are creating novel metal nanoparticles capable of efficient light collection and focusing, in order to enhance the efficiency of light-driven chemical reactions. Utilising the Electron Beam Lithography (EBL) system, thin film deposition and characterisation tools available at MCN, the team have created networks of precisely controlled metal nanoparticles with precision down to 10nm.
Preliminary results indicate that certain nanoparticle arrangements can lead to an enhancement of light-driven decomposition of water pollutants by at least eight times the current rate of decomposition. They have achieved this by creating arrays of nanoparticles using aluminium, an abundant and inexpensive metal, which offers great potential for future deployment in large-scale applications.
The team are currently optimising the shapes and geometrical arrangements of the nanoparticles to achieve optimal rates of photochemical rates. Through this optimisation process they are addressing key fundamental questions, which will provide guidance in the development of processes for creating complex hydrocarbons from carbon dioxide.
You can read more about this project in The Dark Side of Plasmonics, published in Nano Letters.